RADSUM - Topical Workshop on RADiation effects in SUperconducting Magnets
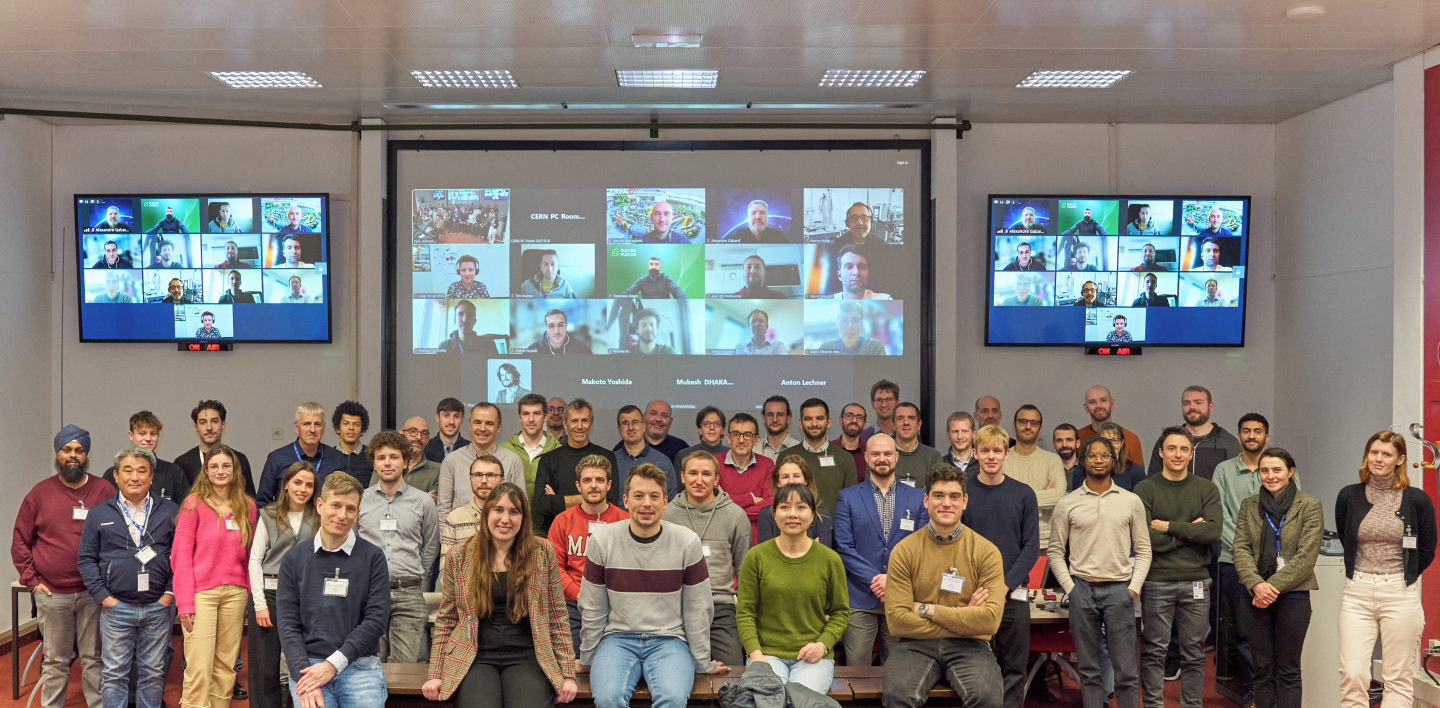
-
Establish an overview of activities and results, and discuss experimental data and modelling work on radiation effects in superconductors, in particular HTS;
-
Quantify and contrast radiation environments for different applications, in particular particle accelerators and fusion facilities;
-
Advance the understanding of radiation-induced degradation of material properties with the goal to establish radiation damage limits for superconductors and other magnet components;
-
Discuss R&D directions for enlarging the material database, and improving the radiation resistance of magnets;
-
Foster synergies among communities (e.g. high-power targetry).
The workshop program includes both invited and contributed talks. Abstract submission is closed.
Registration for in-person participants closes on Dec 20th 2024 (noon).
Practical information for the first day (January 15 2025):
The workshop starts at 2 PM and will take place in the Kjell Johnson auditorium (Building 30, 7th floor, see link for details). You can register and collect your workshop badge between 1:15 PM and 2 PM in the room next to the auditorium (30/7-012).
For those who registered to the magnet hall tour (January 15 2025 at 10 AM), please come to Building 30, 2nd floor, before 9:45 AM (see link for details).
-
-
09:15
→
09:45
Registration (for participants of the magnet hall tour) 30m 30/2-040
-
10:00
→
11:00
Visit to magnet hall 1h 30/7-018 - Kjell Johnsen Auditorium
-
13:15
→
14:00
Registration 45m 30/7-018 - Kjell Johnsen Auditorium
-
14:00
→
14:30
Welcome 30/7-018 - Kjell Johnsen Auditorium
-
14:30
→
16:10
Overview of accelerator and fusion facilities and their radiation environments 30/7-018 - Kjell Johnsen AuditoriumConvener: Simon Chislett-McDonald (Tokamak Energy Ltd)
-
14:30
Radiation challenges for magnets in present and future colliders (HL-LHC, FCC, Muon Collider) 20m
Superconducting magnets in high-energy particle colliders are often exposed to a harsh radiation environment. In many cases, the magnets need to be sufficiently shielded in order to dissipate the radiation-induced heat and to protect equipment against long-term radiation damage. The secondary radiation fields and shielding requirements must be thoroughly studied during the design phase of collider facilities. This contribution gives an overview of present and future high-energy colliders and their radiation environment and highlights the differences between hadron and lepton colliders.
Speaker: Anton Lechner (CERN) -
14:55
J-PARC superconducting magnets and radiation environments 20m
The J-PARC accelerator has been jointly operated by JAEA and KEK since 2007 and a variety of experiments has been carried out using secondary beams of pions, muons, neutrinos and other particles generated by proton beam with a power of several hundreds of kilowatts from 3 GeV Rapid Cycle Synchrotron and 30 GeV Main Ring. Superconducting (SC) magnets have been widely utilized in J-PARC. In particular, the SC magnets for the muon experiments have to be operated under severe radiation environment where a yield of muons is maximized by a combination of high field and intense proton beam bombardment with target nuclei. In this view, development of radiation resistant materials and their experimental validation through the irradiation tests have been carried out. In addition, the magnet design studies considering the degradation of the properties and the production of radioactive nuclei (in particular tritium) during the operation have been conducted.
In this presentation, a general overview of the J-PARC facility and the active and planned SC magnets will be introduced. The dedicated studies on radiation resistance of the SC magnets including the future project will be reported.Speaker: Dr Tatsushi Nakamoto (KEK) -
15:20
The HiMB Project at PSI: Magnets in a high radiation environment 20m
The Paul Scherrer Institute (PSI) operates the world’s most powerful continuous beam proton accelerator, capable of delivering a beam current of up to 2.4 mA with a beam power of 1.4 MW. The proton beam is directed at two carbon targets, generating a large flux of pions and muons. The interaction between the beam and the targets, along with beam scattering, results in a significant radiation load on the surrounding components. The radiation impacts the magnets along both the primary and secondary beamlines. In the context of the IMPACT project, PSI is set to install a new target along with upgraded secondary beamlines. With plans to increase the beam current to a maximum of 3 mA, the radiation load on critical components will remain substantial.
This presentation will introduce the PSI facilities, outline the major challenges encountered in magnet design for their high-radiation environment, and discuss the technical solutions chosen to ensure the long-term operational stability of the new secondary beamlines. It will also focus on the current efforts to install superconducting magnets in the proton accelerator facility in the context of the HiMB project.Speaker: Alexandre Louis Gabard -
15:45
Radiation environment in medical and industrial machines 20m
Superconducting magnets have been used for more than fifteen years in protontherapy systems, starting with NbTi (Varian, IBA) and Nb3Sn (Mevion). These magnets, designed with large temperature, field and current margins, proved to be reliable and show no sign of conductor performance degradation.
The development of HTS presents opportunities and triggers questions about their suitability for medical and industrial applications, such as activation. The purpose of this presentation is to present the radiative environment in medical and industrial applications.
This presentation will provide an overview of the radiation environment around radioisotope production, industrial irradiation and protontherapy or carbon therapy systems. with a focus on the latter. We will depict their main features, which magnet technologies are used and key associated operational characteristics.Speakers: Eric Forton, Vincent Nuttens
-
14:30
-
16:10
→
16:40
Coffee break + group picture 30m 30/7-018 - Kjell Johnsen Auditorium
-
16:40
→
18:10
Overview of accelerator and fusion facilities and their radiation environments 30/7-018 - Kjell Johnsen AuditoriumConvener: Simon Chislett-McDonald (Tokamak Energy Ltd)
-
16:40
Fusion magnets and their radiation environment 20m
Among the key enabling technologies of compact fusion reactors there are high-temperature superconducting (HTS) magnets. Despite the great improvements recently achieved, one of the main challenges that still need to be faced is the evaluation of the impact of radiation in HTS tapes.
To face this challenge, it is crucial to have a comprehensive view of the radiation environment the HTS will be exposed to. This environment strongly depends on the reactor design, so that advanced 3D neutronics simulations are needed.
In this talk we give examples of the radiation environment of superconducting magnets in fusion reactors estimated with Monte Carlo simulations using the PHITS code on 3D CAD-imported models.
This approach yields the expected neutron and secondary particles spectra impinging on the HTS material at the working conditions, the map of the deposited power and information about the damage in terms of displacement per atom (dpa) and Primary Knock-on Atom (PKA) spectra. The output of such simulations can then be used as input for detailed thermal, structural electromagnetic and atomistic damage analysis.
Speaker: Daniele Torsello -
17:05
The radiation environment within the STEP fusion device 20m
The Spherical Tokamak for Energy Production (STEP) is the UK’s major technology and infrastructure programme to build a prototype fusion powerplant that will demonstrate net energy, fuel self-sufficiency and a viable route to plant maintenance.
STEP will use high temperature superconducting (HTS) magnet technology within toroidal and poloidal field (TF and PF) coils to confine the plasma. The radiation environment inside STEP will be extreme, with approximately 5x10$^{20}$, 14.1 MeV neutrons being released from the deuterium-tritium plasma every second. A significant amount of novel shielding is therefore required to ensure magnet lifetimes in the region of several full power years.
Utilising a Monte-Carlo approach, STEP has identified the main driver to lifetime uncertainties as the neutron fluence/damage limit. To reduce this uncertainty, research to better understand the neutron fluence/damage limits will be vital.
This talk will present an overview of the radiation environment in STEP, the current proposed magnet shielding solutions, the magnet lifetime assessment methodology and plans for magnet testing.
Speaker: Tim Eade -
17:30
Radiation Environment of ITER Superconductive Magnets 20m
During operation, ITER superconductive (SC) magnets are subjected to significant thermal loads which mostly result from the deposition of energy by plasma-generated neutrons, as well as from secondary gamma radiation produced when neutrons interact with matter. In addition to thermal loads, total neutron fluence and absorbed dose in key magnets components like the magnet’s insulators should be of concern to every fusion SC magnet designer.
Using for the first time a full-tokamak 360° model, a comprehensive and detailed assessment of the heating deposited during 500 MW DT plasma operation (normal operation) on ITER SC magnets was computed. This allowed to fully take into account the asymmetries of ITER’s geometry and highlight the impact of various components, such as the NBI and the EC Upper Launcher, on the heating patterns of the magnets. Furthermore, the contribution of activated cooling water in the tokamak was also considered and was found to be a substantial source of heating for all the SC magnets, except for the Toroidal Field Coils (TFCs). Some lesson learnt related to effective nuclear shielding for SC magnets in a tokamak environment are also presented.Speaker: Davide Laghi (Fusion For Energy)
-
16:40
-
09:15
→
09:45
-
-
08:30
→
10:35
Modelling of radiation effects in superconductors 30/7-018 - Kjell Johnsen AuditoriumConvener: Daniele Torsello
-
08:30
Radiation effects on HTS: improved pinning vs. pair breaking 20m
The first part of the talk will summarize the physics behind the changes of the superconducting properties upon radiation. The introduced defects enhance flux pinning and scattering of the charge carriers. The implications of these two effects on metallic and high-temperature superconductors will be compared. While the change in pinning is currently difficult to predict, the influence of the increased scattering rate can be modelled by a recently proposed model.
The second part of the talk will outline possibilities of a reliable prediction of the performance change in mixed radiation environments by benchmarking experiments or numerical calculations or combinations of them.Speaker: Michael Eisterer -
08:55
Pinning regimes and modeling of flux trapping relevant to HTS in the radiation environment of fusion reactors 20m
It is crucial to immobilize magnetic vortices threading the superconductors. Capturing vortex pinning from microscopic interactions with defects poses a very difficult yet insightful task. The theory of strong vortex pinning provides the necessary starting point. We revisit the different regimes of strong-pinning theory and investigate them using large-scale numerical solutions of the time-dependent Ginzburg-Landau equations [1-3]. We explore the magnetic-field dependence of the critical current density, jc(B), for superconductors containing defects with different sizes and densities. In a wide parameter range, the vortex configuration is disordered and jc(B) features a power-law decay, where the power index decreases with the particle density. We find a first-order transition of the pinning ground state towards double-occupancy of defects leading to a non-monotonic pin-breaking force and peak effect. Our results provide a framework investigating pinning properties of irradiated materials in fusion applications.
[1] RW et al., Physical Review B 93 064515 (2016)
[2] RW et al., Superconductor Science and Technology 31 014001 (2017)
[3] RW et al., Physical Review B 98 054517 (2018)Speaker: Roland Willa (HES-SO Valais/Wallis) -
09:20
Molecular dynamics simulations of radiation-induced defects 20m
The superconducting properties of rare-earth barium cuprates (REBCOs) are extremely sensitive to the presence of crystal defects. Irradiation of REBCOs with energetic particles produces a plethora of defects, difficult to characterize thoroughly with experimental investigations alone, in particular at the expected working conditions. Atomistic simulations can assist in the understanding of the generated defect structures and provide insights in the microscopic mechanisms leading to change in properties.
In this talk I will show how the radiation damage is modelled from atomistic simulations, focusing on molecular dynamics (MD) simulations of collision cascades. I will start by introducing the typical setup for running MD simulations to model the first stages of defect production induced by a primary recoil, followed by our current understanding of radiation-induced defects in YBa$_2$Cu$_3$O$_7$. Recent progresses in the integration of damage according to experimentally relevant radiation environments by combination of binary collision approximation and MD will be shown, as well as improvements of the description of interatomic interactions with machine learning potentials.Speaker: Davide Gambino (Linköping University) -
09:45
Density functional theory modelling of the defect structures in irradiated REBCO coated conductors 20m
Understanding irradiation damage in high-temperature superconductors is important as new compact fusion reactors rely on these materials to generate the high magnetic fields needed to confine the plasma. The superconductor of choice is (RE)Ba2Cu3O7-δ (RE = rare earth element), REBCO. We have used high-energy-resolution X-ray absorption spectroscopy (XAS) to probe the local environment around the Cu atoms in irradiated REBCO coated conductors as a way of understanding the defects that form. In XAS, a core-electron is excited into the unoccupied states and the resulting spectrum reflects the local environment of that atom. Spectra from all the atoms of an element contribute to the experimental spectrum and interpretation of the edge features resulting from multiple environments can be challenging. Using density functional theory (DFT), we have simulated spectra from a variety of specific atom environments (including a number of defects), which allows us to interpret our experimental spectra in terms of atomistic structures. We find that the differences between spectra from pristine and irradiated materials can be explained by the presence of Frenkel defects in the irradiated sample.
Speaker: Rebecca Nicholls (University of Oxford) -
10:10
First-principles study of defect formation and diffusion in YBa2Cu3O7 20m
High temperature superconducting (HTS) materials, such as YBa2Cu3O7-δ, will be exposed to high energy neutrons that may impact the superconducting properties and ultimately lead to degradation of the magnetic field strength. Density functional theory (DFT) calculations have been used to investigate the energetics of point defects in YBa2Cu3O7. The formation energies of point defects indicate oxygen defects are dominant, followed by Cu defects. Ba and Y defects are less likely to be produced in a radiation environment. The DFT results predict an anisotropic diffusivity of oxygen defects. Oxygen prefers to diffuse on ab planes with a vacancy mechanism, while oxygen could diffuse along c-axis with both interstitial and vacancy mechanisms at relatively high temperatures. In addition, ab initio molecular dynamics simulations of low-energy recoil events in YBa2Cu3O7 are carried out to determine the threshold displacement energies, dynamics of defect generation, and charge transfer during the process. Such computational studies, when coupled with cryogenic neutron irradiation, offer the potential to accelerate the qualification of HTS magnet materials for fusion applications.
Speaker: Li Yang (University of Tennessee, Knoxville, TN, USA)
-
08:30
-
10:35
→
11:00
Coffee break 25m 30/7-018 - Kjell Johnsen Auditorium
-
11:00
→
12:40
Modelling of radiation effects in superconductors 30/7-018 - Kjell Johnsen AuditoriumConvener: Daniele Torsello
-
11:00
Threshold Displacement Energies of Oxygen in YBa2Cu3O7: A Multi-Physics Analysis 20m
High-temperature superconductors (HTS) are promising materials for next-generation fusion reactors and particle accelerators, owing to their remarkable field strengths at elevated temperatures. In these environments, radiation will impinge on the HTS, causing damage. Assessing the extent and nature of this damage is essential for determining the material's lifespan and any necessary shielding requirements.
The threshold displacement energy (TDE) can be used in predictive radiation damage models to predict material damage under radiation fluence. We provide the tools to assess the radiation hardness of YBa2Cu3O7 (YBCO) HTS by calculation of the TDE for all symmetrically distinct oxygen atoms. These results are obtained using an existing empirical potential, which we subsequently validate by a large-scale DFT investigation of TDEs in YBCO. An averaged TDE for the O atoms in YBCO is provided for use in analytical damage models, at temperatures of 25 and 360 K. Compared to previous work, we find decreased TDEs, and propose a number of defect structures that, to the best of our knowledge, have not yet been reported for YBCO.
Speaker: Mr Ashley Dickson (Lancaster University) -
11:25
Present state-of-the-art dpa models 20m
Atomic collision processes are fundamental to numerous advanced materials technologies, such as electron microscopy, semiconductor processing and nuclear power generation. Understanding the atomistic scale phenomena happening during the primary damage production has been a research topic for many decades, both computationally and experimentally. The standard measurement of quantifying the damage is the Norgett-Robinson-Torrens displacements per atom (NRT-dpa) model. This model is known to have several shortcomings, such as the value it gives neither correspond to the real number of defects produced nor the number of atoms that were displaced. In the last decade, there have been several improvements to this dpa calculation. In this talk, several of the newly developed corrections to the dpa calculation will be presented.
Speaker: Fredric Granberg (University of Helsinki) -
11:50
Displacement damage modelling in the FLUKA Monte Carlo code 20m
FLUKA is a general-purpose code for the Monte Carlo simulation of coupled hadronic and electromagnetic radiation showers in complex aterial geometries, accounting for the transport, interaction, and decay (where applicable) of over 60 particle species (photons, leptons, hadrons, and ions) at energies up to the PeV and down to the keV (down to 0.01 eV for neutrons). A wide range of radiation-induced observables can be readily estimated with built-in scoring facilities, including energy/dose deposition, particle spectra, radioactive inventories, to name but a few, rendering FLUKA a versatile tool for a quantitative assessment of radiation-matter interaction problems, particularly for the particle accelerator community.
In this talk, focus will be on FLUKA's approach to displacement damage quantities, especially on displacements per atom (DPA), both in terms of the standard Norgett-Robinson-Torrens DPA and of the recently included athermal-recombination-corrected DPA. FLUKA's coherent treatment of various particle species' contributions to DPA will be discussed, highlighting the constraints imposed by FLUKA's condensed-history account of charged-particle tracks.
Speaker: Francesc Salvat Pujol (CERN) -
12:15
DPA modeling in the PHITS code 20m
To predict the operating lifetime of materials in radiation environments, the displacement per atom(dpa) value, which is the average number of displaced atoms per atom of a material based on the Norgett-Robinson-Torrens (NRT) model, is widely used as an exposure unit and is defined by elastic energy transfer to Primary Knock on Atoms (PKAs), as well as high-energy effects (e.g., nuclear interaction) that results in atomic displacements. Because the NRT-dpa is a simple function of the damage energy of a projectile, it has been incorporated in the Particle and Heavy Ion Transport code System (PHITS). The PHITS code also includes the athermal-recombination-corrected dpa (arc-dpa) model, which is a more realistic model than the NRT model. A comparison of the measured displacement cross sections with the calculated results of the NRT-dpa and the arc-dpa cross sections indicates that arc-dpa provide better quantitative descriptions of the displacement cross section than NRT-dpa.
This presentation introduces the dpa modelling in the PHITS code.Speaker: Yosuke Iwamoto
-
11:00
-
14:00
→
16:05
Irradiation experiments for superconductors 30/7-018 - Kjell Johnsen AuditoriumConvener: Davide Gambino (Linköping University)
-
14:00
Irradiation Studies on HTS Materials in Japan: Results and Future Directions 20m
Irradiation studies on high-temperature superconductors (HTS), particularly REBCO (Rare-Earth Barium Copper Oxide), are critical for understanding their performance in high-radiation environments, such as those found in particle accelerators and fusion reactors. This work focuses on neutron irradiation experiments conducted in Japan, which investigate the effects of neutron fluences of up to 10²² n/m² on the superconducting properties of REBCO materials. These studies are driven by Japan’s efforts to develop radiation-resistant technologies for next-generation scientific infrastructure, including the second target station of the Materials and Life Science Experimental Facility (MLF) at J-PARC. The planned installation of a superconducting pion capture solenoid magnet, designed to withstand radiation levels of up to 100 MGy, underscores the significance of these efforts.
This presentation will provide the current status of HTS conductor irradiation studies, including performance evaluation results and future plans aimed at enhancing the reliability and resilience of HTS materials in extreme radiation environments.Speaker: Dr Mukesh Dhakarwal -
14:25
Proton beam irradiation facility plan at J-PARC and activity on displacement damage study 20m
On 2024, J-PARC achieved the goal of high-power beam operation of 1 MW for the spallation neutron source. The allowable beam power can be said to be determined by the beam intercept materials for the high-power accelerator. J-PARC plans to build a Proton Beam Irradiation facility using 400 MeV protons to study materials, especially beam intercept ones used for the high-intensity proton beam. This facility will be used for a multi-purpose purpose, not only for material damage induced by radiation but also for single-event effects (SEEs) studies of semiconductor devices using proton and neutron for space and terrestrial usage and medical RI production. Also, the activity of displacement damage study, in terms of studying displacement cross-section, will be presented.
Speaker: Shin-ichiro Meigo (J-PARC/JAEA) -
14:50
Neutron irradiation experiments on HTS 20m
The results of two decades of neutron irradiation experiments on high temperature superconducting tapes (coated conductors) will be summarized. The change of the critical current is determined by a competition of improved pinning by the introduction of nanometre sized defects and a reduction of superfluid density by pair-breaking scattering on point-like defects. The latter is directly evidenced by the reduction of the transition temperature and leads to a universal degradation not depending on the particular tape or the type of radiation.
Speaker: Michael Eisterer -
15:15
Ion irradiation experiments at Oxford University 20m
Understanding the effect of irradiation with fast neutrons at cryogenic operating temperature on the properties of REBCO HTS is crucial for the design of magnets for fusion tokamaks. However, suitable high flux neutron sources such as fission reactors are not widely available, performing in situ cryogenic measurements in these reactors is challenging, and neutron irradiation activates the samples making experiments slow and expensive. Ion irradiation offers an alternative fast and widely available route to studying irradiation damage in these materials. In Oxford, we have focussed on using helium ions as a proxy for the point defect damage that neutrons produce throughout the lattice, as it is this damage that results in a drop in transition temperature and ultimately the degradation in current carrying performance. Here we will summarise the experiments undertaken by the team in Oxford over the past few years as part of an EPSRC funded research programme are targeted at answering two questions: do neutrons and light ions produce the same point defect landscapes, and what is the effect of temperature on irradiation damage of REBCO HTS?
Speaker: Susie Speller -
15:40
Cryogenic irradiation of REBCO tapes for fusion magnet 20m
The deuterium-tritium reaction is the most promising source of fusion energy for power plants but generates high-energy neutrons that compromise reactor components - from the plasma-facing walls to fusion magnets. In compact, high-field REBCO-based fusion devices, radiation damage will limit the lifespan. Simulating the fusion environment in test facilities is challenging but essential to predict REBCO tape degradation.
We developed a cryogenic ion irradiation facility to inflict radiation damage at 20 K, the operating temperature of REBCO fusion magnets. We used it to irradiate REBCO tapes with 1.2 MeV protons, while measuring in-situ the superconducting properties. Results show that 20 K irradiations degrade transition temperature, critical currents, and n-value—by ~40% more than irradiations at 300 K, suggesting prior room-temperature studies overestimated the radiation tolerance of REBCO tapes.
To achieve a fusion-like environment, we currently implement at the 6 MW MIT reactor a setup for fast neutron irradiation at 20 K with in-situ transport measurements in fields up to 14 T. In 2025, this facility will provide high-fidelity data on the radiation resistance of REBCO tapes.
Speaker: David Fischer
-
14:00
-
16:05
→
16:30
Coffee break 25m 30/7-018 - Kjell Johnsen Auditorium
-
16:30
→
18:00
Irradiation experiments for superconductors 30/7-018 - Kjell Johnsen AuditoriumConvener: Davide Gambino (Linköping University)
-
16:30
Effect of gamma radiation on HTS - present understanding 20m
HTS magnets employed in fusion plants and high-energy physics experiments will be subjected to significant gamma ray fluxes. Gamma rays ($E_γ$ > 100 keV) have energies orders of magnitude more than the binding energies of REBCO lattice ions ($E_b$ ~ 10s eV) and Cooper-pairs ($E_b$ ~ 10s meV) and are, in principle, therefore capable of altering REBCO microstructure and suppressing superconductivity. The potential of gamma rays to affect the superconducting properties of REBCO has been investigated by researchers since REBCO’s discovery. Unfortunately, the literature is inconsistent, with many papers reporting conflicting results. In this presentation, we delve into this literature (focussing on more recent works), present the general understanding on the effect of gamma rays on REBCO, and suggest directions for future work.
Speaker: Simon Chislett-McDonald -
16:55
Microscopy, spectroscopy and other analytical techniques 20m
The degradation in critical temperature of REBCO coated conductors has been attributed to the generation of point defects, particularly on the oxygen sublattice. This introduces substantial challenges for observing the defects directly because the low mass oxygen atoms are not visible in the most widely used atomic resolution electron microscopy technique, high angle annular dark field imaging (HAADF). Therefore, alternative techniques are required to probe the oxygen defects. Here I will introduce a range of state of the art spectroscopy and microscopy techniques that we have found are sensitive to the point defect damage in REBCO, including high energy resolution x-ray absorption spectroscopy, Raman spectroscopy and electron ptychography.
Speaker: Susie Speller -
17:20
RaDIATE Collaboration overview and achievements in the last few years on material studies 20m
In order to operate reliable beam-intercepting devices in the framework of energy and intensity increase for next generation accelerators, the RaDIATE collaboration (Radiation Damage In Accelerator Target Environment) managed by Fermilab, brings together existing expertise in nuclear material and accelerator targets from 20 international institutions to execute a coordinated strategy for high power targetry R&D. This collaboration is generating new and useful materials data for application within the accelerator and the fission/fusion communities.
I will give an overview of the RaDIATE R&D program and the achievement in the last few years on material studies in support of High Power Targetry development, including results obtained from irradiation test, development of novel materials and the prospective towards future irradiation campaign. An overview of the different facilities used by the RaDIATE Collaborators will be presented (Irradiation stations and Post Irradiation Examination facilities).Speakers: Frederique Landre Ep Pellemoine, Kavin Ammigan
-
16:30
-
08:30
→
10:35
-
-
08:30
→
10:35
Irradiation experiments for organic magnet components 30/7-018 - Kjell Johnsen AuditoriumConvener: David Fischer
-
08:30
Radiation hardness requirements for organic materials in magnets and radiation environments in muon colliders, FCC, HL-LHC 20m
An extensive experience has been gained throughout the last decades in the characterization of the radiation field impacting the magnets of existing and envisaged high-energy particle colliders. Detailed spatial distributions of the dose absorbed by the most exposed coils have been calculated by means of Monte Carlo simulation codes, through the description of the relevant radiation sources and the implementation of the machine model. Maximum values reached over the accelerator operation time could be provided, identifying the nature of the particles contributing the most and determining the effectiveness of different kinds of mitigation measures, aiming at complying with the assumed damage limits.
This contribution will review the present knowledge for the indicated cases, while outlining the methodology applicable to others.Speaker: Francesco Cerutti (CERN) -
08:55
How can we increase the dose limits of organic insulation materials in superconducting accelerator magnets? 20m
In superconducting magnets of particle accelerators like HL-LHC, FCC-hh and a muon collider, shielding is required to reduce the dose absorbed by the magnet materials. A goal of the CERN Polymer Laboratory irradiation study is to increase the acceptable dose limit of the insulation systems beyond the limit of about 30-50 MGy that is presently assumed for shielding design.
Irradiations are performed with 60Co gamma rays, 24 GeV/c protons, and with mixed neutron gamma irradiation at a reactor and a spallation source. To assess the effect of the irradiation temperature, irradiations of identical materials are performed in ambient air, in inert gas, and in liquid helium.
The most important functional property of an insulation system is it resistance against electrical breakdown. Since dielectric failures are often caused by prior mechanical failures of the insulation system, the irradiation induced degradation of the mechanical properties of the insulation system is a main concern. Methods for determining dielectric and mechanical irradiation damage threshold values will be discussed.References
[1] https://www.mdpi.com/2073-4360/16/9/1287
[2] https://www.mdpi.com/2073-4360/16/3/407Speaker: Christian Scheuerlein (CERN) -
09:20
Main results of the polymer irradiation studies for the ITER project at ATI Vienna 20m
The talk summarizes the results of the combined neutron and gamma irradiation studies on polymers performed for the development of the insulation system of the ITER PF coils. The ultimate tensile stress (UTS) and the interlaminar shear strength of epoxy raisins and cyanate ester based polymers were determined as a function of the neutron fluence or dose rate. The fatigue behaviour of the UTS was assessed as well. It was found that cyanate ester based polymers are much more robust against radiation than epoxy resins, but a blend of the two materials was decided to be the best compromise between the high radiation hardness of the cyanate esters and the easier handling of the epoxy resins during the impregnation process.
Speaker: Michael Eisterer -
09:45
Dosimetry Techniques and Radiation Test Facilities for Radiation Damage Studies 20m
This contribution aims to review basic dosimetry principles, give examples for dose calculations as well as a brief overview of the available irradiation facilities for material irradiation studies and their associated dosimetric means. Their interest, limitations and some practical aspects involving the organization of irradiation campaigns for total ionizing dose testing will be also discussed together with potential opportunities for future irradiation experiments on materials at CERN.
Speaker: Federico Ravotti (CERN) -
10:10
Cryogenic cooling options for radiation testing 20m
Low temperature environments are vital for radiation testing of materials used in accelerator components. The cryogenic environment can either be established by immersion in a cryogenic fluid like Helium or in a dry manner by using cryocoolers as cooling source. The operation of helium cryostats requires frequent refill via long distance transfer lines. A direct cooling with cryocooler platforms is not in all cases possible due to the high level of radiation and possible degradation and activation of materials in the cryocooler and its vicinity. Novel concepts of He circulation loops cooled by the cryocooler at some distance would overcome such limitations. The presentation will compare existing tests stand from CERN and introducing the novel concept of remote cooling loops.
Speaker: Dr Torsten Koettig (CERN)
-
08:30
-
10:35
→
11:00
Coffee break 25m 30/7-018 - Kjell Johnsen Auditorium
-
11:00
→
12:30
Design of superconducting magnets and shieldings for high-radiation environments 30/7-018 - Kjell Johnsen AuditoriumConvener: Luca Bottura (CERN)
-
11:00
Design of COMET capture solenoid and shielding system 20m
As accelerators become more powerful, superconducting magnets used to generate and transport secondary particles such as muon sources are also required to have high radiation resistance. The COMET experiment at J-PARC requires the highest intense muon beam to search for rare processes beyond the Standard Model. The muon beam line is designed with high-field superconducting solenoids to capture pions and muons emitted from the production target. Although the target is surrounded by thick radiation shield, the magnet is expected to be exposed to severe radiation of neutrons and other particles from the target. The material in the magnet is carefully chosen to withstand in the high radiation environment. Especially irradiation effects in the stabilizer of the superconducting cable and heat transfer in the superconducting coils have been studied by performing series of irradiation tests. The design of COMET capture solenoid and shielding system will be described.
Speaker: Makoto Yoshida -
11:25
Magnet shielding design in present and future colliders 20m
Magnet shielding design in high-energy particle colliders is a multidisciplinary challenge, requiring consideration of diverse constraints to ensure both performance and longevity of components. An integrated design shall address key factors such as power load management in relation to quench and cryogenic limits, dose tolerance for organic materials, displacement per atom (DPA) thresholds for conductor materials, and the impact of shielding thickness on magnet aperture requirements. Using the Muon Collider as a primary example, along with insights applicable to the High-Luminosity Large Hadron Collider (HL-LHC), design considerations for balancing these requirements effectively are outlined. Shielding material choices are further evaluated considering several aspects such as their efficiency, structural and engineering implications, cost, and thermal management requirements.
Speaker: Daniele Calzolari (Universita e INFN, Padova (IT)) -
11:50
Research and development of ceramic-insulated HTS magnets for high-radiation environments 20m
A construction plan for a second target station of Materials and Life Science Experimental Facility (MLF-TS2) has been proposed at J-PARC. At the MFL-TS2, which extracts a primary proton beam with a power of approximately 1 MW, a superconducting solenoid for a muon source is placed at the backward position particle generation target to maximize muon beam yield. The nuclear heating would be an extremely challenging for the superconducting solenoid: the absorbed dose of the solenoid reaches 100 MGy and the magnet system has to be operated under the heat load 450 W.
KEK has been performing research and development of mineral-insulated superconducting magnet based on REBCO conductors for the TS2 muon sources solenoid. The REBCO tapes, 4 mm wide and 40 m long, have been uniformly formed with an electrically insulating layer of about 0.03 mm thick by ceramic coating on both sides. Several prototype coils have been fabricated using the ceramic-coated conductors by wet-wound method with a commercial ceramic adhesive. In this contribution, the current status of ceramic coating on conductors and coil fabrication and the preliminary results of performance evaluation tests will be presented.Speaker: Masami Iio
-
11:00
-
14:00
→
16:05
Design of superconducting magnets and shieldings for high-radiation environments 30/7-018 - Kjell Johnsen AuditoriumConvener: Luca Bottura (CERN)
-
14:00
Efficient Magnet Designs and Radiation effects for PSI 20m
The Paul Scherrer Institute (PSI) operates the world's most powerful proton accelerator. Currently, all magnets are resistive and require about 2.6 MW of power. Due to the high level of radiation, about 20% of the magnets are designed to be radiation resistant.
Energy efficiency is nowadays a crucial concern for the accelerator community, prompting an exploration of alternative solutions to resistive magnets. Superconducting magnets could be a viable, energy-efficient alternative for both primary and secondary beamlines of large accelerator facilities.
To investigate this possibility, a superconducting demonstrator magnet based on ReBCO coils is planned to be placed in an irradiated environment representative of the machine. The aim is to study the effect of radiation both on the HTS conductor itself and on all the magnet components surrounding it. A first measurement of ambient radiation in the PSI accelerator was performed on two HTS tapes and we present the results. In parallel, the conceptual design of a superconducting Nb-Ti transport solenoid to be installed in the new HiMB secondary muon beamline was realised and the impact of radiation on the operating point was simulated.Speaker: Ciro Calzolaio -
14:25
Design of magnet and shielding systems for DEMO 20m
Designing magnet systems for fusion devices, including tokamaks, stellarators, and mirror machines, requires careful consideration of the intense radiation environments in which these systems operate. This study focuses on the conceptual design and optimization of magnet systems for DEMO and VNS machines, with particular attention to achieving a balance between compactness, durability, and performance, alongside effective radiation shielding. Multiple cable configurations, such as High-Temperature Superconductors (HTS) in Cable-in-Conduit and Dry-Conductor designs, are analyzed to determine optimal solutions for magnetic field strength, neutron shielding efficiency, and thermal stability. Key design considerations include coil sizing for maximum plasma confinement, management of neutron heat load and cumulative radiation dose on superconducting materials, and the implementation of shielding strategies to mitigate radiation-induced degradation. Through comparison of various design options, this work identifies configurations that enhance the operating lifetime and stability of magnet systems under high neutron flux conditions.
Speaker: Dr Lorenzo Giannini (EUROfusion) -
14:50
Design of magnet and shielding systems for compact fusion machines 20m
Recent progress in high temperature superconducting (HTS) magnets has greatly enhanced the potential of spherical tokamaks (ST) for harnessing fusion energy. Given the compactness of an ST and high plasma density, the centre column shield is subjected to challenges such as high neutron load, volumetric heating from neutrons and gamma radiation, and a limited amount of available space. The selected material must exhibit exceptional neutron and gamma attenuation for ensuring magnet lifetime, good thermo-mechanical properties for heat removal, with minimal radioactive waste.
A combination of python scripts and Monte Carlo radiation transport codes have been utilised to select optimal materials based on their shielding properties for different neutron and photon energy ranges. For STs, a combination of Tungsten Borides or Carbides are used to shield high energy neutrons. Once the materials were selected, a combination of high-fidelity unstructured mesh neutronics calculations and FEA analyses are used to assess conceptual shield designs for shielding efficacy as well as heat management of the shield.
Speaker: Gurdeep Kamal (Tokamak Energy Ltd) -
15:15
A look toward ARC: Lifetime conditions for REBCO in compact fusion power plants 20m
Commonwealth Fusion Systems (CFS) is building the world’s first compact net energy fusion device, SPARC, by utilizing recent advances in the commercial production of 2G REBCO coated conductors. SPARC’s delivery and operation will inform the design of ARC, the world’s first fusion power plant, scheduled to come online in the early 2030s. The design, safe operation, and quench protection of high field fusion magnets requires the detailed characterization of the field (B), angle (Θ), and temperature (T) dependence of the critical current (Ic) of these tapes at the operational range for each magnet system, which is in the range of 20K, 20T. However, in a fusion power plant, the superconducting tapes will be subjected to neutron irradiation over the plant lifetime. Therefore, the impact of the neutron irradiation on the electromagnetic and mechanical properties of the coated conductors during operation must also be well understood and designed for. This talk focuses on summarizing the relevant conditions expected in ARC that the tapes will see to guide future studies on irradiation of coated conductors for compact fusion applications.
Speaker: Ashleigh Francis (Commonwealth Fusion Systems) -
15:40
Explorative energy deposition studies of the superconducting dipoles of the C-ion gantry for CNAO 20m
In the context of the EuroSIG project, a carbon-ion gantry is being designed for possible installation at CNAO (the National Center for Oncological Hadrontherapy located in Pavia, Italy) and MedAustron (located in Wr. Neustadt, Austria). The current lattice design foresees superconducting (SC) dipoles and normal-conducting quadrupoles. Differently from the normal-conducting ones, SC magnets are sensitive to local beam losses, which lead to local energy deposition and potential quenches, thus inducing considerable downtime of the gantry.
This contribution presents explorative studies of energy deposition in the SC dipole magnets presently foreseen for the EuroSIG C-ion gantry. The studies are carried out by means of Monte Carlo simulations, using the FLUKA code to characterise ruling factors like beam spot size. In this exploratory phase, simulations were carried out considering simplified beam impact conditions.
Together with key results, the magnet geometry is briefly presented and implications on the gantry design drafted.Speaker: Ms Giulia Tosetti (CNRS)
-
14:00
-
16:05
→
16:30
Coffee break 25m 30/7-018 - Kjell Johnsen Auditorium
-
16:30
→
17:30
Guided discussion - need for new data, theories and experiments 1h 30/7-018 - Kjell Johnsen AuditoriumSpeakers: Michael Eisterer, Susie Speller
-
08:30
→
10:35